John Muir’s theories on the formation of Yosemite were derided by the day’s scholars, who considered him an “ignoramus”. However, studies into the geology of Yosemite prove that most of Muir’s theories were right on the dot. Not bad for a self-described “unknown nobody”.
Guide to Yosemite
Geology of Yosemite
Most Park Junkies are aware that the Yosemite we admire today was formed by glaciers and their effects on the underlying granitic rock of the Sierra Nevada Mountains. However, the history of this fantastic landscape begins far before the massive glaciers ever reached this area. This landscape was formed long ago, by extreme forces, far below the Earth’s surface.
The beginnings of Yosemite are much the same as those of its neighboring national parks to the south, Sequoia and Kings Canyon. The Sierra Nevada is America’s longest mountain range, stretching some 400 miles from north to south. This mountain range is home to all three aforementioned parks, and the formation of these mountains laid the foundation upon which glaciers would later act.
A Collision of Plates
During the Cretaceous Period, between 66 and 140 million years ago, continental shifting was well underway. The ancient continent of Pangea was completely fractured by this point, and global landmasses were ever-so-slowly beginning to take the shapes that we see today. Large tectonic plates were moving due to molten activity far below the Earth’s surface, growing oceans and reshaping continents.
As the great North American Plate was pushed westward, its course collided with a series of smaller plates, including the ancient Farallon Plate, which was being forced eastward by the massive Pacific Plate.
Subduction
Over the course of tens, if not hundreds of millions of years, these plates were slowly forced below the North American Plate in a process geologists refer to as subduction. Subduction creates immense, unfathomable pressure and friction, and also introduces oceanic waters to subterranean depths and existing molten temperatures within the Earth’s mantle.
Many theories purport that this water acts to lower the melting temperature of subterranean rock, while at the same time creating highly pressurized pockets of steam. Temperatures in excess of 1832 ℉ (1,000℃) melt rocks and creates what we call magma.
Magma, being less dense than surrounding solid rocks that were not subjected to the same pressure, was forced upward. Some reached the surface, where it became lava, and cooled quickly. Most of this magma however, remained buried within the Earth’s upper crust, allowed to cool slowly there.
Portions of this buried rock would be forced upward by a combination of volcanic and subductive forces, and would reach the surface forming an upheaval in the earth. The majority however would remain buried, not to see the light of day for the next 60-90 million years, and most of it has still not been unearthed.
Igneous Rock
Rock that is formed by fire in such a manner is known as igneous rock. It is a result of the cooling and subsequent solidification of molten rock material. (The term comes from the Latin, ignis – meaning fire.)
There are two types of igneous rock: plutonic and volcanic. Both are formed from magma, however volcanic igneous rock is formed above the Earth’s surface where it cools quickly and forms lightweight rocks with extremely small crystals that are only visible by microscope.
Plutonic igneous rock, on the other hand, solidifies while under pressure beneath the surface, where it cools slowly. This leads to the formation of much denser rock, with larger crystals that can be easily observed by the human eye. Since this rock is formed in the underworld, it is named for the Roman god Pluto, mythical ruler of the underworld.
Yosemite’s stone landscape consists mainly of plutonic igneous rocks, mainly in the form of granite. Granite is not simply an igneous rock, but a more complex, intrusive igneous rock. This means that numerous types of rocks “intruded” one another while in liquid from, creating the complex array of colors and shapes evident in the park’s granite terrain.
The Sierra Nevada Batholith
These rocks, the result of extreme heat created by plate tectonics, slowly formed a massive batholith that stretched more than 400 miles from north to south below the Earth’s surface, known as the Sierra Nevada batholith. A batholith is an extremely large collection, or composite of individual plutonic rocks. (The term originates from the Greek words bathos, deep, and lithos, rock.)
It is worth noting that the Sierra Nevada batholith is an extremely complex geologic formation. Geologists have mapped more than a hundred individual masses of plutonic rock in the area surrounding Yosemite and hundreds more still in the Sierra’s entirety.
Emplacement of such rocks was taking place as numerous volcanic eruptions were occurring directly above, spewing lava upon the Earth’s surface. Such activity had been taking place in this area for an untold amount of time. Metamorphosed volcanic remnants are today exposed in the Sierra that date to the Triassic Period, more than 200 million years ago.
Violent episodes of volcanic activity likely occurred in this area throughout the late-Jurassic and Cretaceous Periods, as volcanic rocks found near Mount Dana and in the Ritter Range date to between 100 and 118 million years.
By the beginning of the Cenozoic Era, about 66 million years ago, the magmatic influences of the subduction zone leveled off, perhaps due to the subducted Farallon plate and other fragments leveling off beneath the continental plate, thus moving the zone of magma generation eastward.
Ancestral Sierra Nevada
Nevertheless, left behind was a linear mountain range, running parallel to the edge of the North American Plate. This range is known as the ancestral Sierra Nevada. It was not at all what we see today.
Erosion has always been a constant force on our planet and was partly responsible for the unearthing of the Sierra Nevada batholith. As these ancient mountains grew, they eroded. For the next 40 million years, this range would shrink faster than it would grow.
Some geologic reports estimate that this range attained elevations of up to 13,000 feet by the end of the Cretaceous Period, 66 million years ago, only to be eroded to a rolling upland with a topographic relief of only two to three thousand feet by the beginning of the Miocene Epoch, some 25 million years ago.
Then it all changed. Sometime between 15 and 25 million years ago, a major change in the movement of the continental plates occurred. The Farallon plate was completely consumed below the continent, and another plate slid it to take its place… but this massive plate was moving in a totally different direction.
A Meeting of the Plates
At this point, the Pacific plate, the world’s largest, and the North American plate, the world’s second largest, came into direct contact for the first time. The Farallon plate’s easterly movement had imposed an inward compression, or a “push” on the continent, which is generally associated with thrust faulting, while the new plate, with its northwesterly direction, exerted an outward, extensional force, or a “pull”.
This directional shift led to a myriad of effects, not the least of which was the formation of the San Andreas fault, born at the junction of the two major plates. Now the Pacific plate would essentially “slide” along the North American plate, which, as we know today, produces those wonderful California earthquakes…
More importantly for Yosemite however, was the effect this had on the Sierra Nevada batholith, as the outward pull exerted by the Pacific plate’s movement had far-reaching consequences.
Basin and Range
To the east of the Sierra Nevada Mountains lies the Owens River Valley, and to the east of that, a series of jagged mountains and wide, flat valleys running north to south that extends across the state of Nevada and into western Utah. This is known as the Basin and Range Province.
The Basin and Range is not limited to this area, it is indeed, huge. Within its boundaries are parts of the states of Idaho, Wyoming, Nevada, Utah, Arizona, Texas and even a large section of Mexico. As previously stated, this is a land of mountains and valleys, or in scientific terms, horst and graben.
The unique structure of the Basin and Range is evident as one gazes toward the east from high atop one of the Sierra range’s towering mountains.
The vertical relief from the summit of the Sierra at Mount Whitney (14,505’) to the Owens Valley floor (3,556’) is more than 11,000 feet. The eastern side of this valley gives rise to the Panamint Mountains which separate the Sierra, and the highest elevation in the Lower 48, from the lowest elevation in the western hemisphere at Badwater Basin (-282’), in Death Valley National Park. The distance between these two extremes is less than 100 miles.
Further to the east from this vantage point, the visible topography is one of vast contrast, with north to south running mountains and valleys stretching as far as the eye can see. If one is to continue the drive east across the great state of Nevada, the repetition will continue, all day…
Thinning Lithosphere
Some scientists believe the Basin and Range may be evidence of a “pull” from the Pacific plate. The horst and graben topography typified in this province is evidence of a lithographic expansion from east to west.
Basically, the Pacific plate is slowly pulling the subterranean world westward, effectively thinning the lithosphere, which includes the Earth’s crust and upper mantle. Subsequently, mountains are separated and flat, sunken valleys, (grabens) are left between the peaks, (horsts).
The exact effect that this may have had upon the Sierra is unclear and is today a subject of some controversy in the geologic community. However it is obvious that the Sierra Nevada Batholith is undoubtedly, an uplifted block of the Earth’s crust, with a severe vertical relief on its eastern edge, and a long westward slope leading to the Central Valley in the west.
It is possible that the “pull” from the Pacific plate has thinned the lithosphere under these landmasses and that such pull has allowed the rising mountain range’s western slope to effectively sink, while the westward movement of the Basin and Range may one day help to explain the Sierra’s more pronounced eastern uplift. Meanwhile research and debate regarding the recent uplift of the Sierra continues.
Early Geologic Study
While the exact causes of the dramatic rise of these mountains remain unclear, the effects of water, gravity and glaciation upon their heights is well known.
Serious scientific study of the Yosemite area began with an 1863 excursion by the Geological Survey of California, headed by the State Geologist, Josiah Whitney, accompanied by William Brewer and Charles Hoffman. The group visited Yosemite Valley and explored the “High Sierra” as Whitney referred to the area’s high country.
Although Whitney and his party acknowledged evidence for the action of glaciers in the area, it seems they underestimated, or completely dismissed the extent to which these large bodies of ice affected the formation of the Sierra, with Whitney ascribing the origin of Yosemite Valley to a cataclysmic collapse of the valley floor.
John Muir
Soon an opposing theory surfaced. In 1869, John Muir, a self described “unknown nobody” appeared in the valley. He took up residence in the Yosemite Valley, and began to wander deep into the mountains, seemingly exploring each individual rock and examining every detail of the natural landscape.
Within a year of his arrival, the “unknown nobody” had concluded that the sheer granite walls and polished domes of the high country were not the result of some “grand cataclysm”, as described by Whitney, but were instead the product of the “eternal grind” of massive glaciers over thousands of years.
Whitney, by now a professor of geology at Harvard University, was not impressed with the musings of a “mere sheepherder” and someone he derided as “an ignoramus”. He would continue to advocate his cataclysmic collapse theory until his death in 1896.
In short order, Muir’s glacial theory would be proven. In 1871, while wandering about in the upper regions of the Sierra, he came upon a “living glacier”, the first of sixty-five he would eventually find. He produced this evidence to the geologic community, and led renowned geologists of the day to the glacier, who then began to accept the glacial theory.
Studies soon began into the extent of glaciation in the Sierra and in time, findings revealed that while Muir’s theory was indeed correct, it was likely an overestimation of the extent to which the glaciers acted upon the landscape surrounding Yosemite.
Muir had proposed that ice once covered the entire Sierra Nevada and had continued to the Central Valley, and beyond. Scientific studies initiated in the early 1900s came to dispute that hypothesis, finding that while glaciers were responsible for much of the scenery we enjoy today, Muir’s proposal was likely exaggerating the effect that ice played on the surrounding areas.
Jointing
To understand the role of glaciation on the granitic rock of Yosemite, it is important to briefly mention the role of “jointing” in the massive Sierra Nevada batholith.
As covered earlier, a batholith is a large collection of buried rocks. Under the immense the pressures directed upon them within the Earth’s surface, these rocks coalesce, or consolidate, into massive singular bodies and often appear to be uniform upon unearthing by erosion or uplift. Nevertheless, weakness often exists within the large bodies of stone.
During tectonic movements, or during tilting of the batholith, enormous strains are placed upon these massive stone structures. Such stresses sometimes result in deep fractures, or cracks within the batholith. These cracks are apparent on the surface of rocks throughout Yosemite, they are referred to as joints, and the are essential in the formation of the landscape evident here today.
Joints form long, linear depressions, which often become channels for erosive elements such as water, air, weathering, and eventually, glaciers. Over the course of hundreds of thousands of years, even a dense stone such as granite will succumb to the weakening effects of these forces and what is originally a small fracture may one day become a major drainage, such as Yosemite Valley.
Various types of joints are found throughout Yosemite and depend greatly upon the grain and texture of the rocks in which they form.
Generally, the more siliceous, or quartz-rich, rocks, such as granite and granodiorite, are less susceptible to fracture and jointing than less siliceous rocks such as tonalite and diorite. It is also true that these finer grained rocks will suffer fractures, or joints, in closer proximity than the coarser grained stones. Combined, these weaknesses allow these types of rock to succumb to the effects of erosion more quickly.
A great example of the difference between these types of rock can be easily witnessed in Yosemite Valley.
Rockslides
Just to the west of El Capitan is an area known as the Rockslides, where a massive pile of broken stone fragments lies separated from the cliffs above. So many stones lie here that height of the talus pile reaches nearly three-quarters of the way up the wall. This jumbled collection of stone consists mostly of diorite, a fine-grained stone and the least siliceous of the plutonic rocks in Yosemite Valley.
Conversely, just to the east rises the 3,200 foot vertical face of El Capitan, which is comprised mostly of El Capitan and Taft Granites, two of the most siliceous plutonic rocks in the valley. The difference is striking. El Capitan stands tall and largely unjointed and unbroken, and the talus pile at its base is small.
Once the process of jointing had created a passage for water, it was only a matter of time. Slowly, over the course of millions of years, water worked its magic. Pebble by pebble, it dismantled stone structures that we can imagine, stood tall and formidable. However, the continuous flow of water is the most formidable force of all. The Yosemite we witness today is the result of this continuous flow of water, in both liquid and solid form.
As the Sierra Nevada batholith began to uplift more rapidly in the late Cenozoic Era, water-flow over its ancient drainages became more forceful and violent. As the area’s topographic relief grew, so did the effects of water upon the rugged terrain.
Water
Today we find deeply incised, yet unglaciated canyons downstream from Yosemite. The Merced River Valley, just west of El Portal, and the Tuolumne River both hold examples of such water-carved canyons. These canyons lie beyond the reach of the glaciers that John Muir concluded were responsible for the formation of much of the Yosemite landscape.
The fact that these rivers formed such canyons beyond the reach of glaciers indicates that the forces of water had pronounced effects on the valleys of Yosemite prior to the periods of glaciation that scoured the landscape and polished its peaks. The courses of such streams and rivers were likely inherited from drainage patterns that existed prior to the more recent batholithic uplift.
Over the course of tens of millions of years, water found its way down from the heights of the Sierra Nevada range, during both the range’s original uplift, and during its more recent uplift. The effects of water flow in the range left deep V-shaped valleys.
Although water was indeed responsible for much of the initial erosion that led to the formation of Yosemite, glaciers alone hold responsibility for the unique U-shaped valleys that we witness today.
Glaciers
Glaciers are formed when some of the snow that falls during one winter remains through the following summer and becomes part of the following winter’s snow base. Thus, heavy winter snowfalls, or cool summer temperatures, or both, contribute to the accumulation of snow.
If snowfall continues to outpace snowmelt for an extended period of time, perhaps only a few centuries, such accumulation will grow to the point that layers of snow in the lower regions of the deposit are compacted into ice by the sheer weight of the snow above.
At a thickness of about 100 feet, the ice begins to spread outward, unable to support its own weight. As this outward flowing ice meets outlying upward slopes, it begins to move, or flow, downhill. At this point, a glacier is born.
The study of glaciers is often limited because evidence of older glaciers is often destroyed by subsequent glaciers. Glaciers act as giant bulldozers, pushing sediment and debris in front of them as they creep down gorges, leaving the piles of debris behind upon their retreat. Such piles of debris are referred to as the glacier’s terminal moraine.
Generally, it is only if a younger glacier is smaller than an older glacier, that the older glacier’s existence can be confirmed and studied. However, if a younger glacier is larger than its predecessors, there is rarely adequate terminal moraine evidence to build theories surrounding the earlier body of ice. Thus it is nearly impossible to conclude with any degree of certainty how many glaciers have covered a given area of glacial impact via such study.
While there exists various theories as to how many periods of glaciation had effect on the Sierra Nevada, scientists can conclusively agree upon the presence of at least three glacial periods of significant influence in Yosemite: Tioga, Tahoe, and Pre-Tahoe. There may be more, but well dude, we just don’t know…
The Tioga Glaciation
The Tioga glaciation is the youngest of the known glacial periods, beginning about 30,000 to 60,000 years ago. During this time, a cooling climate witnessed the growth of small glaciers in high cirques that were formed by earlier glaciers. The young glacier grew steadily, projecting outward and downward, slowly encompassing all in its path.
At the apex of its growth, some 15,000 to 20,000 years ago, the Tioga glaciation covered all but the highest peaks and divides of the northern and eastern Yosemite. Its ice flowed down into valleys, completely filling Hetch Hetchy, however barely reaching Bridalveil Meadow in Yosemite Valley. The tallest rocky arêtes and horns in the high country peaked above a massive ice-fields where today we find smooth granite cliffs, alpine lakes, lush flowery meadows and conifer forests.
Upon retreat of the Tioga glaciation from the Yosemite Valley, a large lake formed that consumed much of the valley floor. This lake, like all that form upon the retreat of glaciers in the Sierra, was to be but a temporary body of water, as the Merced River would wash away the lower lying moraine which would eventually drain the lake.
The Tahoe Glaciation
Prior to this, an even more massive system of ice was at work in the mountains of this park. The earlier Tahoe glaciation’s moraines extend past those of the Tioga in most areas of Yosemite, however, this period’s glaciers never quite reached the extent of the later glaciation in Yosemite Valley, as there is little evidence of the Tahoe glaciation in the valley. This would indicate an earlier period of glaciation, or periods of glaciation, that must be responsible for the massive landmark at the heart of the park.
Most of the evidence for the glaciation(s) that produced the U-shaped contour of Yosemite Valley, the pre-Tahoe glaciation, lies above the valley floor in the form of strangely perched rocks known as erratics. These out-of-place stone statues often stand out from the natural surrounding as they are mounted atop areas where they don’t belong, usually with the erratic protecting the erosion of the underlying area, giving it a more impressive mantle upon which to stand.
There may have been many more episodes of glaciation here, but these three are the only agreed-upon periods, although the dates to which they can be attributed are vague and the extent of the pre-Tahoe glaciations are largely unknown.
Nevertheless, the theory of the “unknown nobody”, John Muir stands true, glaciers are indeed responsible for the Yosemite that we see today and who knows, perhaps the ice monsters will one day return to once again, further mold the granite walls of the magnificent Yosemite Valley…
Guide to Yosemite
Relevant Links
National Park Guides
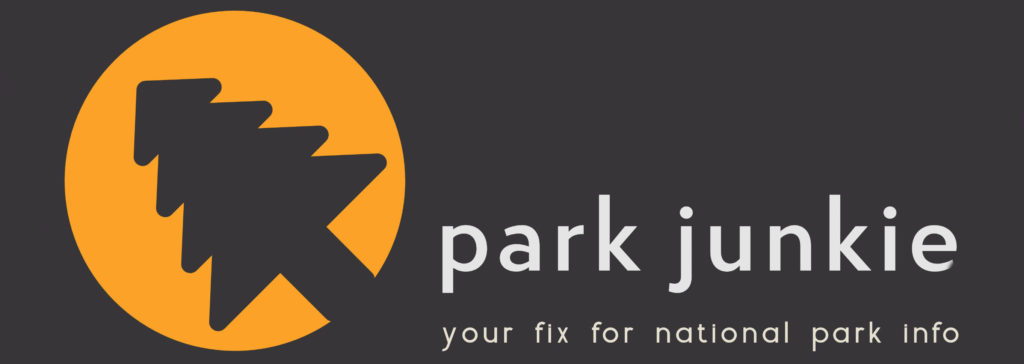
All content found on Park Junkie is meant solely for entertainment purposes and is the copyrighted property of Park Junkie Productions. Unauthorized reproduction is prohibited without the express written consent of Park Junkie Productions.
YOU CAN DIE. Activities pursued within National Park boundaries hold inherent dangers. You are solely responsible for your safety in the outdoors. Park Junkie accepts no responsibility for actions that result in inconveniences, injury or death.
This site is not affiliated with the National Park Service, or any particular park.